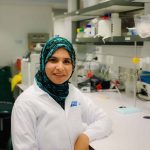
Farha Ramzan
Research Fellow
Personalised and evidence-based dietary approaches are crucial for promoting optimal health and well-being for each individual.
This is why we should gain more knowledge about our unique genetics to understand how our body digests and metabolises certain food and nutrients.
Nutrigenomics is only the start
In recent times, nutrigenomics, a scientific field investigating the interaction between genetics, nutrition, and health, has received considerable interest due to its capacity to transform personalised nutrition and preventive healthcare.[1]
The potential of nutrigenomics lies in customising dietary guidance according to an individual’s genetic profile.
Nonetheless, it is essential to acknowledge that its effectiveness in isolation is limited without incorporating complementary scientific disciplines such as:
- molecular biology
- bioinformatics
- clinical research
The interplay between nutrition and gene expression becomes more intricate due to the involvement of genetics, epigenetics, gut microbiota, and environmental factors.[2]
Nutrigenomics as a stand-alone approach has limited benefits
Limited genetic data availability affects personalised dietary precision, and complex gene-nutrient interactions hinder clear causality, leading to uncertain results.[3] Therefore, a holistic approach involving lifestyle, socio-economic factors, and cultural influences is essential.
Recognising what limits nutrigenomics is vital for evidence-based, safe, and effective personalised diets, enhancing overall well-being.
That is why integrating genetic data with clinical observations, studies, and trials may help validate personalised dietary recommendations.
Dangers of One-Size-Fits All Diet
Nutrigenomics is vital in bringing attention to the hazards of a One-Size-Fits-All diet approach. What may be beneficial for one person could be harmful to another, potentially leading to nutritional deficiencies or adverse effects.
By uncovering the influence of genetic variations on individuals’ responses to specific nutrients and dietary patterns, nutrigenomics emphasises the necessity of personalised nutrition.[4]
Below are some of the examples of different compounds found in common food and beverages that can affect individuals differently.
Caffeine
Coffee, a widely consumed caffeinated beverage, is primarily metabolised by the cytochrome P450 1A2 (CYP1A2) enzyme.
Genetic variation (rs762551) in the CYP1A2 gene has been found to impact caffeine metabolism.[5] Those with the AC and CC genotypes (slow variants) are considered slow metabolisers, while individuals with the AA genotype are regarded as fast metabolisers of caffeine.
On an average the half life of caffeine is 4-6h in most adults, but if you are a slow metaboliser it could be as long as 6-12h. However, it is not yet known to what degree caffeine metabolism is altered between fast and slow metabolisers.
Iron
Iron plays multiple crucial roles in the body, being a component of cytochrome heme and serving as a key molecule in hemoglobin and myoglobin heme, which binds oxygen in red blood cells.
However, excessive iron absorption can harm the body. Genetic variation in the HFE gene (C282Y), responsible for iron uptake, is shown to raise iron absorption and accumulation, possibly causing overload and health issues.[6]
Vitamin D
Vitamin D intake is an important preventive measure for osteoporosis and overall health improvement. However, the effectiveness of supplementation can vary significantly among individuals.
Notably, genetic variation in the Vitamin D Receptor gene (VDR), is shown to exhibit different responses to vitamin D supplementation, potentially influencing an individual’s ability to absorb and utilise vitamin D from sunlight or dietary sources.[7]
RELATED — Vitamin D: The sunshine hormone for stronger bones
Vitamin B12
Vitamin B12, or cobalamin, is a vital water-soluble micronutrient essential for maintaining human health.
Genetic variations can influence the tissue status of vitamin B12 by affecting the proteins involved in its absorption, cellular uptake, and intracellular metabolism.
For instance, Methionine Synthase (MTR) gene (rs1805087) is shown to impact the utilisation of vitamin B12 in the body.[8]
Calcium
Calcium, the most abundant mineral in the human body, plays a crucial role in various biological functions.
Genetic variation in the calcium-sensing receptor (CASR; A986S) is reported to influence its absorption and utilisation, thus affecting the calcium levels in the body, leading to several bone and mineral metabolism disorders and cardiovascular disease mortality.[9]
Vitamin A
Vitamin A, an essential fat-soluble micronutrient, regulates vision, immune responses, and normal development.
There are two main forms of vitamin A in the human diet: preformed vitamin A, and provitamin A carotenoids, mainly as β-carotene.
Genetic variations rs12934922 in the β-Carotene 15,15′ oxygenase 1 enzyme (BCO1) gene have been found to influence serum β-carotene levels, eventually reducing vitamin A levels in the body.[10]
Vitamin B9
Folate, a vital B-complex vitamin in our diet, plays a significant role in DNA synthesis and maintaining methylation reactions within cells.
Polymorphisms in the genes responsible for folate metabolism can potentially disturb folate status in the body. One frequently encountered variation involves the methylene-tetrahydrofolate reductase (MTHFR) gene.[11]
Variants in the MTHFR gene have been implicated as genetic risk factors for developing various diseases, including congenital heart diseases and breast cancer.
Sodium
Sodium is an important nutrient vital for normal body function and overall health.
A normal range of 3-5g/day of dietary sodium intake has been associated with the lowest cardiovascular disease risk. With the risk of cardiovascular disease increasing if the sodium >5g/day or falls <3 g/day.
Sodium is an important electrolyte
Polymorphisms in the Sodium Channel Epithelial 1 Alpha (SCNN1A) gene, a subunit of the epithelial sodium channel (ENaC) gene responsible for the clearance of sodium in the body, has been reported.[12]
This polymorphism may impact sodium reabsorption in the kidneys, potentially influencing an individual’s sodium sensitivity and ability to regulate blood pressure.
Vitamin E
Alpha-tocopherol, a crucial vitamin E form, acts as a potent lipid-soluble antioxidant in the human body.
Regulated by the alpha-tocopherol transfer protein (TTP), alpha-tocopherol acts as a physiological defence against conditions related to oxidative stress, including ageing.
Genetic variations in TTPA gene have been observed among different individuals, impacting their vitamin E levels, affecting antioxidant defence and susceptibility to oxidative damage.[13]
Catechins
Catechin, are the major components of green tea and are believed to contribute to its beneficial health impacts.
The beneficial effects of green tea are believed to stem from the strong antioxidant characteristics of its primary catechin, epigallocatechin gallate (EGCG).
UDP glucuronosyltransferase 1A4 (UGT1A4) enzyme is shown to play an important role in metabolising EGCG.[14] However, polymorphism in the UGT1A4 can is reported to affect its activity, potentially leading to variations in how individuals metabolise EGCG from green tea.
Saturated fat
Saturated fat is a type of dietary fat that is found in various animal and plant sources.
Saturated fats are an essential component of a balanced diet and play roles in hormone production and cell membrane structure. Excessive consumption has been linked to health concerns.
The Apolipoprotein E gene (APOE) gene has been shown to regulate its metabolism in the body. However, individuals with the polymorphism in the APOE4 allele might metabolise the fat differently, thereby having an increased risk of heart disease when consuming a diet high in saturated fats.[15]
What are Genotype and Phenotype, and why are they important?
Genotype and phenotype are two fundamental concepts in genetics and biology that play a crucial role in understanding the inheritance of traits and the development of various characteristics in living organisms, including humans.
Source: Young, A. Deconstructing the sources of genotype-phenotype associations in humans. (2019)
Genotype
Genotype refers to an individual’s genetic makeup, representing the specific combination of genes inherited from their parents.[16]
This genetic information is encoded within the DNA, which serves as the blueprint for an organism’s structure and function.
Genotype encompasses all the different forms of a gene, known as alleles, found at specific positions on the chromosomes. These alleles can be identical (homozygous) or different (heterozygous) for a particular gene.
For example, in humans, the gene for eye colour has different alleles that can result in various eye colours, such as blue, brown, or green. An individual’s genotype for eye colour may be homozygous for brown eyes (having two identical alleles for brown eyes) or heterozygous (having one allele for brown eyes and another for blue eyes).
Phenotype
Phenotype refers to an individual’s observable traits and characteristics that result from the interaction between their genetic makeup (genotype) and environmental influences.[16]
These traits encompass a wide range of features, including:
- physical attributes (e.g., eye colour, height)
- physiological functions (e.g., metabolism, enzyme activity)
- behavioural traits (e.g., intelligence, personality)
The expression of phenotypes is not solely determined by genetics and environmental factors also play a significant role. Factors like:
- nutrition
- lifestyle
- exposure to toxins
- social interactions
can influence how genes are expressed and how phenotypes are manifested.
Linking genotype and phenotype is vital in various fields. This connection is pivotal in nutrigenomics, involving multiple genes, their interactions, and dietary/environmental effects.[17]
Integrating genetic information with dietary and lifestyle factors would allow for personalised nutrition recommendations that have a potential to optimise overall health and well-being.
How to start with a personalised DNA diet
Starting with a personalised DNA diet involves several steps to understand an individual’s genetic makeup and tailor their dietary recommendations accordingly.
Genetic/DNA testing
To understand how an individual’s diet can affect them, it is essential to consider how their genetic makeup may influence their metabolic response.
Genetic or DNA testing plays a crucial role in this process, as it allows the analysis of an individual’s DNA to identify specific genetic variations or mutations that may influence their response to:
- nutrients
- nutrient metabolism
- food allergies
- other relevant dietary factors
Genetic/DNA testing typically involves collecting a saliva or cheek swab sample, which is then analysed to reveal insights into an individual’s genetic traits.[18]
To ensure that the data generated from these tests is analysed accurately and interpreted appropriately, it is crucial to seek the guidance of a qualified healthcare professional, such as a genetic counsellor or dietitian.
Lifestyle, cultural preferences and health goals
When devising personalised DNA diets, it is also crucial to consider not just an individual’s genetic makeup, but also their lifestyle, cultural preferences, and health objectives.
Although genetic information provides valuable insights into how certain nutrients interact with the body, it is not the sole determinant of dietary needs. Factors such as an individual’s physical activity level and daily routines significantly determine their nutritional requirements.[19]
For example, an active lifestyle may require more energy and specific nutrients to support exercise and recovery, while a sedentary person may require a different approach.
Moreover, cultural practices and traditions profoundly influence dietary habits. Therefore, healthcare professionals must design plans considering an individual’s cultural food preferences and dietary norms.
Doing so makes the individual more likely to feel comfortable and motivated to follow the dietary recommendations. Furthermore, personalised DNA diets should be tailored to support an individual’s specific health goals.
Whether the objective is:
- weight management
- cardiovascular health improvement
- managing chronic conditions
- enhancing overall well-being
the recommended diet plan should align with these aspirations.
Progress monitoring
A personalised DNA diet plan must be practical and attainable, considering an individual’s lifestyle and cultural preferences.[20]
By aligning the diet plan with the individual’s daily routines and food choices, they are more likely to stay committed and motivated.
Monitoring is key to success in a DNA diet
Healthcare professionals should schedule periodic consultations to assess the individual’s progress. These check-ins help ensure that the dietary plan continues to align with the individual’s evolving needs and goals.
Adjustments can be made to the DNA diet based on the individual’s progress, challenges, and any new genetic insights that emerge.
Related Questions
1. What are some nutrigenomics foods?
Nutrigenomics does not categorise foods into specific “nutrigenomics foods”.
Rather, it focuses on the understanding that an individual’s unique genetic makeup can influence how certain foods/diets could interact with our body. Some examples would include:
- Cruciferous vegetables (broccoli, cauliflower, cabbage, and brussels sprouts)
- Omega-3 fatty acids (fatty fish, such as salmon, mackerel, and sardines)
- Leafy greens (spinach and kale)
- Berries
- Whole grains (quinoa, brown rice, and oats)
- Beverages, such as green tea.
2. Where can I do a nutrigenomic test?
Nutrigenomic testing has gained huge momentum over the years. Nevertheless, the research behind these tests is still in its infancy.
It’s always best to consult with a health professional specialising in nutrigenomics about getting a referral to a reputable testing facility that uses the polygenic approach of understanding diet-gene interactions.
Important note
It’s crucial to approach personalised DNA diets cautiously and seek guidance from qualified healthcare professionals.
By taking a holistic approach, healthcare professionals can create dietary plans that are both effective and sustainable, leading to improved health outcomes and greater compliance with the recommended dietary changes.
Farha is a Researcher at Liggins Institute, the University of Auckland. Her research interests involve understanding the complex interplay between the genetics, epigenetics and dietary patterns of an individual and how these interactions predispose an individual to develop metabolic diseases.
Currently, Dr Ramzan is working on projects aimed at establishing New Zealand based plants as functional foods.
References
(1) Mathers, J. C. (2017). Nutrigenomics in the modern era. In Proceedings of the Nutrition Society (Vol. 76, pp. 265–275). Cambridge University Press.
(2) Ferguson, J. F., Allayee, H., Gerszten, R. E., Ideraabdullah, F., Kris-Etherton, P. M., Ordovás, J. M., … Bennett, B. J. (2016). Nutrigenomics, the microbiome, and gene-environment interactions: New directions in cardiovascular disease research, prevention, and treatment. Circulation: Cardiovascular Genetics.
(3) Juma, S., Imrhan, V., Vijayagopal, P., & Prasad, C. Prescribing personalized nutrition for cardiovascular health: Are we ready? , 7 Journal of Nutrigenetics and Nutrigenomics 153–160 (2014). Karger Publishers.
(4) Bordoni, L., & Gabbianelli, R. (2019). Primers on nutrigenetics and nutri(epi)genomics: Origins and development of precision nutrition. Biochimie.
(5) Mahdavi, S., Palatini, P., & El-Sohemy, A. (2023). CYP1A2 Genetic Variation, Coffee Intake, and Kidney Dysfunction. JAMA Network Open. Retrieved from https://jamanetwork.com/journals/jamanetworkopen/fullarticle/2800839
(6) Infanti, L., Leitner, G., Moe, M. K., Pehlic, V., Benkert, P., Cattaneo, M., … Buser, A. (2022). Indices of iron homeostasis in asymptomatic subjects with HFE mutations and moderate ferritin elevation during iron removal treatment. Blood Cells, Molecules, and Diseases. Retrieved from https://www.sciencedirect.com/science/article/pii/S1079979622000468
(7) Wong, H. L., Seow, A., Arakawa, K., Lee, H. P., Yu, M. C., & Ingles, S. A. (2003). Vitamin D receptor start codon polymorphism and colorectal cancer risk: Effect modification by dietary calcium and fat in Singapore Chinese. Carcinogenesis. Retrieved from https://academic.oup.com/carcin/article/24/6/1091/2390446
(8) Surendran, S., Adaikalakoteswari, A., Saravanan, P., Shatwaan, I. A., Lovegrove, J. A., & Vimaleswaran, K. S. (2018). An update on vitamin B12-related gene polymorphisms and B12 status. Genes & Nutrition.
(9) Zagrodna, A., Ksia̧żek, A., Słowińska-Lisowska, M., & Łaczmański, Ł. (2020). Calcium-Sensing Receptor Gene Polymorphisms (CASRV1 and CASRV2) and the Physical Activity Level of Men in Lower Silesia, Poland. Frontiers in Genetics.
(10) Suzuki, M., & Tomita, M. (2022). Genetic Variations of Vitamin A-Absorption and Storage-Related Genes, and their Potential Contribution to Vitamin A Deficiency Risks Among Different Ethnic Groups. Frontiers in Nutrition.
(11) Hiraoka, M., & Kagawa, Y. (2017). Genetic polymorphisms and folate status. Congenital Anomalies.
(12) Yang, Y. J., Kim, J., & Kwock, C. K. (2018). Association of Genetic Variation in the Epithelial Sodium Channel Gene with Urinary Sodium Excretion and Blood Pressure. Nutrients. Retrieved from https://www.ahajournals.org/doi/10.1161/HYPERTENSIONAHA.121.18513
(13) Ulatowski, L., Ghelfi, M., West, R., Atkinson, J., Finno, C. J., & Manor, D. (2022). The tocopherol transfer protein mediates vitamin E trafficking between cerebellar astrocytes and neurons. The Journal of Biological Chemistry.
(14) Acosta, L., Byham-Gray, L., Kurzer, M., & Samavat, H. (2022). Hepatotoxicity with High-Dose Green Tea Extract: Effect of Catechol-O-Methyltransferase and Uridine 5’-Diphospho-glucuronosyltransferase 1A4 Genotypes..
(15) Griffin BA, Walker CG, Jebb SA, Moore C, Frost GS, Goff L, Sanders TAB, Lewis F, Griffin M, Gitau R, Lovegrove JA. APOE4 Genotype Exerts Greater Benefit in Lowering Plasma Cholesterol and Apolipoprotein B than Wild Type (E3/E3), after Replacement of Dietary Saturated Fats with Low Glycaemic Index Carbohydrates. Nutrients. 2018 Oct 17;10(10):1524. doi: 10.3390/nu10101524. PMID: 30336580; PMCID: PMC6213759. Retrieved from https://www.ncbi.nlm.nih.gov/pmc/articles/PMC6213759/
(16) Orgogozo, V., Morizot, B., & Martin, A. (2015). The differential view of genotype–phenotype relationships. Frontiers in Genetics. Retrieved from https://www.ncbi.nlm.nih.gov/pmc/articles/PMC4437230/
(17) Wang, F., Zheng, J., Cheng, J., Zou, H., Li, M., Deng, B., … Kan, J. (2022). Personalized nutrition: A review of genotype-based nutritional supplementation. Frontiers in Nutrition. Retrieved from https://www.frontiersin.org/articles/10.3389/fnut.2022.992986/full
(18) Mullins, V. A., Bresette, W., Johnstone, L., Hallmark, B., & Chilton, F. H. (2020). Genomics in Personalized Nutrition: Can You “Eat for Your Genes”? Nutrients.
(19) Vesnina, A., Prosekov, A., Kozlova, O., & Atuchin, V. (2020). Genes and eating preferences, their roles in personalized nutrition. Genes.
(20) Brennan, L., & De Roos, B. (2021). Nutrigenomics: lessons learned and future perspectives. The American Journal of Clinical Nutrition. Retrieved from https://www.sciencedirect.com/science/article/pii/S0002916522006141
Very interesting read!